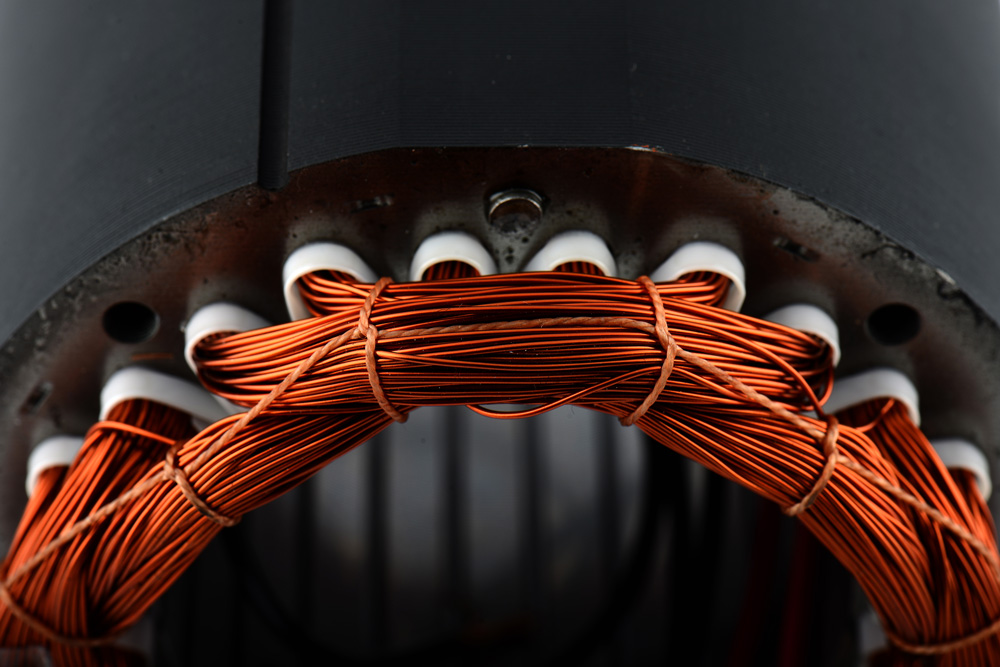
Over the last 150 years, development of the electric motor has gone in fits and starts. Initially, all electric motors used direct current (DC). But with the advent of alternating current (AC) and amid the “battle of the currents” between industrial development giants George Westinghouse and Thomas Edison, Nikola Tesla unveiled his induction motor, a new design that converted the power of alternating electrical current into rotational torque.
It was a game changer. As well as helping Westinghouse win the battle of the currents, it was relatively easy to make, efficient and, crucially, needed no slip rings or brushes to transmit electrical current to the shaft. These parts were the Achilles’ heel of the DC and slip-ring type AC motor, requiring regular maintenance to replace them as they wore out.
Instead, eddy currents and their associated magnetic field were induced in a stack of steel laminations attached to the shaft by the new layout of distributed winding embedded in the stator using the rotating magnetic field the alternating current created. The magnetic field of the stator interacted with the induced magnetic field of the rotor, turning it at nearly, but not quite, the same speed. The asynchronous AC motor was created. The combination of high starting torque and low maintenance saw it quickly adopted for applications that were previously the realm of steam, water, belts/pulleys and horsepower. The reluctance motor, using magnets embedded in the rotor, was also invented in the 19th century. It was handicapped by low efficiency when driven directly from the AC supply. This would change at the end of the 20th century.
The 1970s & 1980s
Fast forward almost a century and the induction motor, universal in its applications on pumps, fans, blowers, machine tools, mixers and other applications, was taken for granted. Manufacturers had refined production methods to reduce costs, sometimes at the expense of efficiency. Its only continuous failing was that it ran at the speed determined by the number of magnetic poles in the winding and the frequency of AC supply. That speed was not to be altered, other than by creating a complex stator winding with two and four or four and six poles embedded in it.
Some plant users overcame this challenge by using eddy current and fluid couplings mounted between the motor and driven machine to adjust the output speed down and up to suit the immediate requirement. However, these couplings were expensive and bulky. On pumps, they added size, complexity and extra maintenance. Belts and pulleys of different diameters could be used to permanently deliver a different pump speed—higher or lower than the motor speed—to suit a continuous application.
Variable Speed Drives
The invention and mass manufacturing of electronic variable speed drives (VSDs) began in the 1960s and 1970s. Industrial pioneers developed several methods to convert the mains electrical supply at its constant frequency (50 or 60 hertz [Hz], depending on geography) to a variable frequency on the output, resulting in a variable speed induction motor driving the pump or fan. With a suitable control system, plant speed could be adjusted to suit a variable load.
The first generation of VFDs was expensive, large and unreliable. They would regularly trip themselves out to self-protect. Operators responded by often demanding that a bypass system of the VFD to the motor be engineered in case of failure. This made them even more costly, bulky and complicated. Thirty years later, VFDs were smaller, affordable and reliable. The size reduction allowed them to be decentralized and mounted locally.
OEMs of pumps took a step further in the 1990s and integrated them into their products and, in the years since, have added self-adjusting smart speed controllers and web connectivity. This simplifies the design and install of hydronic systems while also delivering more reliable outcomes. The pump controllers’ capabilities often include accurate measurement of flow rate and pump generated head—information that can help to deliver higher process efficiency. A benefit of these improvements has also been the removal of bypasses, rendered redundant just as the starting handle became for cars and automobiles after electric self-starters were introduced.
Potential side effects of VFDs, such as harmonic disturbance to the power supply network and electric currents generated within the induction motors traveling through their shaft bearings, are still not fully understood in many industries, leading to some over-engineering and waste of materials and money.
Motors From the 1980s Onward
The move to more efficient motors started in the 1980s. Using more active materials and tighter manufacturing tolerances, their higher efficiency products created a new market and drove regulators to create standards that differentiated the new from the old. Today’s National Electrical Manufacturers Association (NEMA) and International Efficiency (IE) ratings are the results of that initiative. But improvements beyond IE3 and NEMA Premium required motor technology that would not need the induction of currents in the rotor to create the rotor’s magnetic field. The era of the permanent magnet motor was on the horizon. The question was: in which direction would it go?
Permanent magnet motors—surface mount and interior mount
In motor rotors fitted with permanent magnets, the stator winding no longer has to expend power inducing magnetism in it. But how are the magnets best configured in or on the rotor? As their names imply, surface permanent magnet (SPM) motors have magnets on the surface of the rotor and interior permanent magnet (IPM) motors have them embedded inside the rotor, shaped to suit the drive application.
SPM motors deliver good starting torque, but the arrangement of the magnets generates back EMF in the stator at speed, reducing efficiency. SPM motors are speed-limited due to the mechanical limitation of the attachment of the magnets to the shaft. However, this does not apply at the low speed of centrifugal pumps compared with those required for automotive applications.
An IPM motor, embedded in the rotor, allows the shape of the magnets to be optimally configured so the lines of magnetic flux reduce the back EMF at high speeds. Also, no mechanical speed limits apply as they do to SPM.
Reluctance motors—synchronous reluctance
A reluctance motor has specially shaped empty slots in the rotor. If the stator field is correctly aligned to the slots, the rotor will rotate in a low reluctance state, producing reluctance torque. This motor type is low cost, robust, generates high torque and is tolerant of supply faults and overload. The downside is that it has high torque ripple: a fluctuation in torque generated as the magnets react with the stator windings at certain angles during rotation.
Reluctance motors—switched reluctance
This motor type is low in cost, efficient, robust, generates high torque and is tolerant of supply faults and overload. The downside is it also has high torque ripple and produces more noise and vibration.
The SYN RM IPM motor
Placing PM in the slots in the synchronous reluctance motor produces the IPM Syn RM motor. It does not generate back EMF when, at speed, the rotor is aligned to the stator field at one angle and at startup, another angle that generates high torque. One other benefit is that the motor shaft runs cool, improving bearing life. The SYN RM IPM motor uses both reluctance torque and magnetic torque, managing their complementary properties to give high torque at startup with high efficiency and smooth operation at speed. In addition, the power factor is improved. Also, this type requires less magnetic material than a conventional IPM motor.