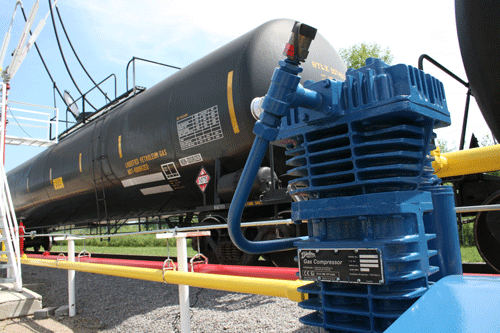
Compressor technology has a crucial purpose, though it only achieves its desired effect if placed in the proper applications. Even then, the proper amount of study and consideration must occur before deciding if those compressors will function better than other technologies.
Simply put, there are no cookie-cutter solutions for any applications that involve the handling and transfer of industrial gases. Therefore, making assumptions based on past experience, or what the other guy is doing, can only get users in trouble.
To help ease the burden of selecting the proper compressor technology for the handling of industrial gases, this article will lay out a general framework that can be used to help the end user identify and select the proper solution for all their different applications.
Know Your Application
When an end user calls and says they have an application where they need to move some product, be it ammonia to
vinyl chloride, the first job of the equipment supplier’s application engineer, and their overriding priority, is asking the most obvious question: Is this even a compressor application?
The operator must define the application and explain what needs to occur through the product-transfer process. After establishing the operation parameters, the application engineer will know if a compressor is an appropriate technology for use in completing the process. There are typically three product-transfer-based applications that will fit into the sweet spot for compressors:
Vapor recovery: This process involves capturing gases that remain in a storage vessel that would, in years past, have been vented to the atmosphere. Today, these gases must be recovered due to stricter environmental and safety regulations, as well as their economic value. Vapor recovery is typically used for liquefied gas vapors left in a vessel post transfer, natural gas vapors in stock tanks, sulfur hexafluoride in electrical transformers, seal leakage from process compressors in larger plants and the emptying of storage vessels prior to their maintenance, reconditioning or replacement.
Pressure boosting: This process consists of moving a gas from one location to another, accomplished by boosting the gas pressure to a level that allows it to be sent to another storage-tank location or process for use at that higher pressure. A simple example is a nitrogen-boosting system that lifts the pressure of nitrogen gas stored at 125 pounds per square inch gauge (psig) (8.6 barg) to a boosted pressure of 300 psig (20.6 barg) to meet process demands. Pressure boosting can also be used for transferring refined natural gas from a low-pressure distribution line into a storage tank that feeds a burner in a heat-treating process. Compressors excel in the pressure-boosting process because they can handle a wide range of pressure-boosting conditions.
Liquefied gas transfer: This operation generally takes place during the loading, unloading or transloading of railcars and can involve both liquid transfer and vapor-recovery applications. Common products handled at this stage can include propylene, carbon dioxide, a large range of refrigerants, propane and a host of other liquefied gases. Basically, almost any liquid that is stored under its own vapor pressure in pressurized tanks is a candidate for the liquefied-gas transfer or vapor-recovery processes.
Know Your Conditions
Once the actual process is identified, the application conditions must be determined. To ease this process, many equipment suppliers use a data sheet they give end users to complete. General questions include site location and elevation, equipment location and whether the equipment is stationary or mobile. The questionnaire will also ask for more specific operational parameters, such as:
- required inlet and discharge pressures and temperatures
- ambient temperature range
- atmospheric pressure (determined by the site elevation)
- electrical-area classifications, such as National Electrical Manufacturers Association (NEMA) 1, 4, 7, 9 or 13
- operational noise levels that can be allowed (generally specified by a maximum decibel level)
- a gas analysis of the product being handled (including the molecular weight and molar percentage of the gas or liquid products being handled)
- required flow capacity
- number of production cycles per day. High-duty cycles will generally require the compressor to run continuously, or on some type of load/unload cycle.
- on-and-off cycle times and their duration/frequency
- types of control switches
- what safety switches and alarms are required to protect the equipment (compressor) and the process
Once these operational parameters are identified, they can be sent back to the application engineer who will select the proper compressor. However, this does not mean they will have a definitive answer. In many cases, the operational parameters might indicate that more than one size or type of machine could be safely recommended for the job.
But this does not mean that there is a one-size-fits-all solution available. It pays to reiterate that every technology and every model design within that technology has concrete limits on what its operational capabilities are. This can also require some outside-the-box thinking. For example, if an application requires a flow rate of 500 standard cubic feet per minute (scfm) at a pressure of 800 pounds per square inch (psi), a single compressor may not be able to meet that standard, but if it is deployed in a series or parallel with a second compressor, that might be the best way to meet the operational parameters.
Surveying the Field
Reciprocating piston compressors are positive displacement machines, as are their diaphragm cousins. There are also positive displacement rotary compressors, such as the vane, screw, liquid ring and blower types. Finally, there are axial or radial turbo compressors. All excel in their own operational niche within the world of industrial-gas transfer, whether it be the ability to produce high flow at high pressure, high flow at low pressure, low flow at low pressure or low flow at high pressure. Again, the operational parameters of the process will provide a roadmap to the best compressor technology.
Another factor is cost. Reciprocating piston compressors are among the most cost-effective technologies available to the market. Still, the operation’s process parameters and requirements will play a large role in the final cost of the equipment required for the application. For instance, machines that must meet American Petroleum Institute (API) 618 design requirements will have a higher purchase price than a non-API 618 design.
While many systems will be able to use a standard machine as is, once the proper one is selected, there will always be some applications in which a specially designed or configured machine is required. In this case, there are bound to be added system costs as the compressor and/or system are modified to fit the needs of the operation.
Narrowing the Focus
Even if a reciprocating piston compressor is the best choice for the operation, there are still other considerations to be made before making a final decision.
Within the family of reciprocating piston compressors there are oil-free, nonlube, lubricated and oil-less designs. Again, knowing the operational parameters and requirements will create a path that leads to the selection of the proper technology. For example, the transfer of crude natural gas might not require an oil-free compressor. The gas itself already has impurities, so small amounts of oil carryover from the compressor will not harm the product or the process.
Emission-compliance requirements, however, could mean that an oil-free design might still be the best solution due to its leak-control capabilities.
Additionally, oil-free compressors are normally more expensive due to the elevated technical requirements of the oil-free design. An oil-free machine will usually always be required if the gas purity of the user’s process gas must not be contaminated by the compressor. These application requirements tend to dictate the need for an oil-free compressor.
Growing environmental considerations might also lead to the more oil-free compressor selection and usage. Some lubricated compressor designs that require oil lubrication in the upper cylinder and valve area do not have a gas-sealing section (distance piece). This can be prone to higher leakage rates, which can be an environmental hazard. With the increased scrutiny by regulatory agencies to fugitive emissions and leaks, operators must be aware of the repercussions. These can include fines and even shutdowns if their operations continue to run afoul of any regulatory mandates.
If the choice is an oil-free reciprocating piston compressor, the actual environmental operating conditions must also be considered. Oil-free designs have specific discharge-temperature limitations. In this instance, the top end of the machine is designed to operate without lubrication, so it has nonmetallic wear parts (e.g., piston rings, packing seals, etc.) that become sacrificial wear parts because they operate without any lubrication.
By design, those parts will need to be serviced and replaced on a regular basis. Typically, this is done as an annual preventative maintenance program. In some applications where operating temperatures are low and operating pressures and compressor rotations per minute (rpm) are in a moderate range, a user can see the compressor operate for a few years without any need for significant maintenance. In other applications where temperatures are high and pressures more demanding, the operator might only get 2,000 hours of service out of the compressor before it needs to have those top-end wear parts serviced. Note that this situation typically occurs in applications with very high discharge temperatures driven by the application’s overall compression ratio. These possibilities must be communicated to the operator on the front end of the selection process to minimize complaints or recriminations on the back end.
Finally, and most importantly, the performance of the reciprocating piston compressor must meet or exceed the requirements of the process. Often, this can mean that the compressor manufacturer will need to reach out to other professionals in the field—distributors, fabricators, system integrators, etc.—for assistance in completing a turnkey gas-compressor system for the application. In the end, everyone involved has the same result in mind: getting the job done reliably, efficiently, safely and cost-effectively.
Reciprocating piston compressors, like any comparable technology, are only effective in performing their duties if they are put in a position to be successful. Reaching a state of ideal operation can be attainable if the proper legwork is done to ensure the compressor can satisfy the many unique and varied needs of the application. By asking the right questions, performing the required research and working closely with the user and other channel partners, the compressor selection process will provide an optimized operation.