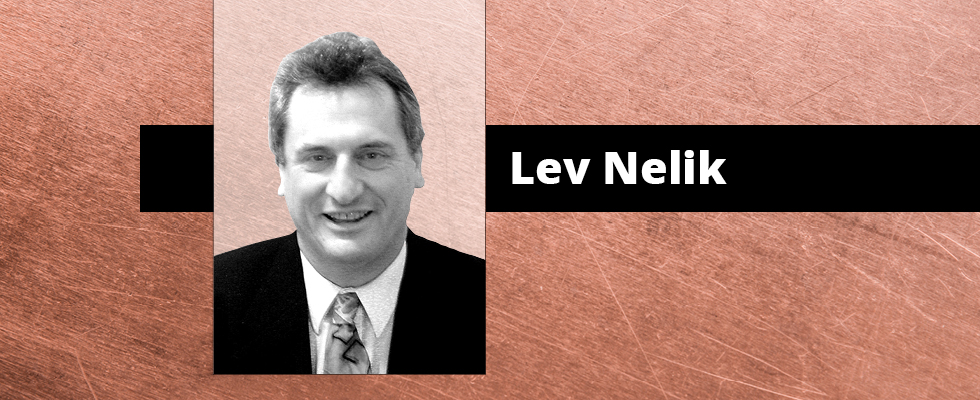
There are two fundamental issues with pumps operating not at, or near to, best efficiency point (BEP): low reliability (things just break), as well as excessive energy consumption (somebody pays much more).
Reliability impact—low efficiency, high radial loads, noise, vibration—becomes a real problem when that happens. Damage to the seals, bearings, shaft, couplings and poor reliability are a direct result of such operation.
But let’s explore the effect of such operation on pump efficiency, and we will estimate wasted energy. The larger the pump, the more energy is wasted when a pump operates off-peak. A full range of American National Standards Institute (ANSI) pumps, for example, as offered by a pump manufacturer, may consist of many sizes to span a wide range of flows, with larger sizes reaching more than 4,000 gallons per minute (gpm).
Image 1 is an overall hydraulic coverage chart. For each size, a head-capacity curve at the maximum and minimum impeller diameter is plotted (sometimes minimum diameter is not shown in order to make the chart less cluttered). This allows us to make an approximate selection of a pump size, and then to look up the individual detailed hydraulic performance curve for that size to finalize the details.
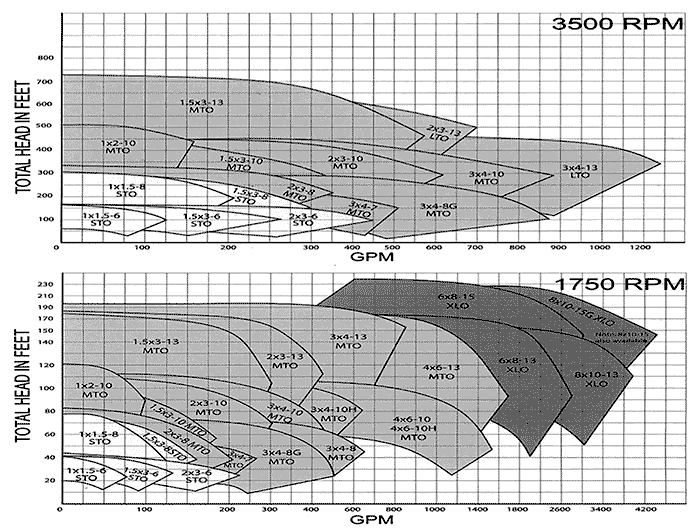
First, consider a case of a relatively small pump. For example, if a pump user is looking for equipment to pump 40 gpm at 140 feet of head, a 1x1.5-6 pump size (with approximately 6-inch impeller diameter) would be selected. The pump will work, but unfortunately will not be operating at its optimum design point.
As evident from the hydraulic curve for this pump size (Image 2), this pump will have 40 percent efficiency (yellow circle). Its design point (red angle), however, is at 58 percent efficiency. The pump operates to the left of its BEP point for the impeller diameter required to achieve the desired head.
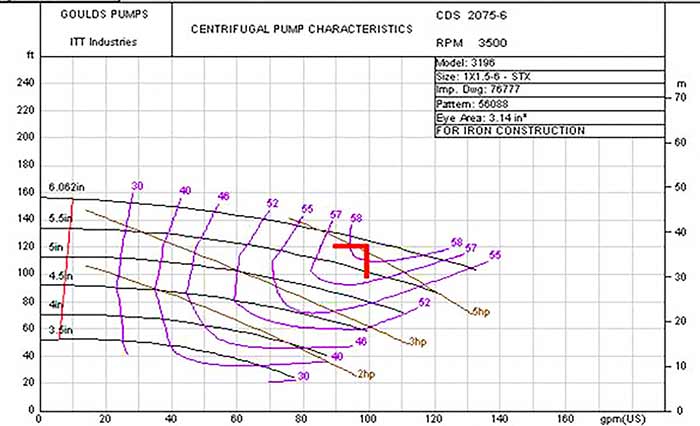
In this example, note that a horsepower (hp) line passes near the operating point at approximately 4 hp, which is roughly 3 kilowatts (kW). How much does it cost to operate this pump if running continuously, 365 days per year, at, say $0.07 per kilowatt-hour?
3 (kW) x 24 (hr/day) x 360 (days/year) x 0.07 ($/kW-hr) = $1,814
Equation 1
Now, what would it cost if the efficiency was somehow improved to the same 58 percent that this pump enjoys when operating at the design point? Obviously, if a pump runs more efficiently, it will take less power. In fact, the power (and thus cost) would be inversely proportional to efficiency:
1,814 x (40/58) = $1,251
The net savings would thus be 1,814 – 1,251 = $563.
Equation 2
This cost may not be too significant for a small pump example like this.
Next, let’s consider a somewhat larger pump (Image 3). Say we have a 4x6-10H size operated at 600 gpm and producing 100 feet of head.
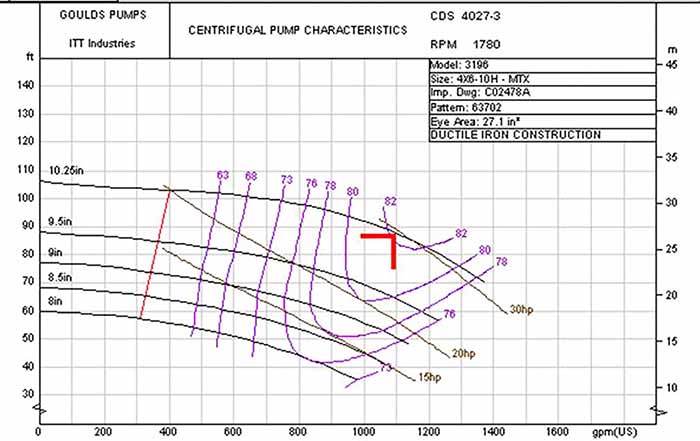
Again, the pump is off the efficiency peak. It operates at approximately 65 percent, whereas its peak efficiency at that diameter (10.25 inches) could be 82 percent. Now, the energy dollars become more pronounced. Its power consumption is approximately 25 hp (19 kW), according to hp lines in the proximity to operating point.
19 x 24 x 360 x 0.07 = $11,491
Equation 3
But it would be less if efficiency was restored to the designed 82 percent, about 21 percent in this case.
11,491 x (65/82) = $9,108
The savings would be: 11,491 – 9,108 = $2,383
Equation 4
Let’s next take an even larger size (Image 4): 8x10-17.
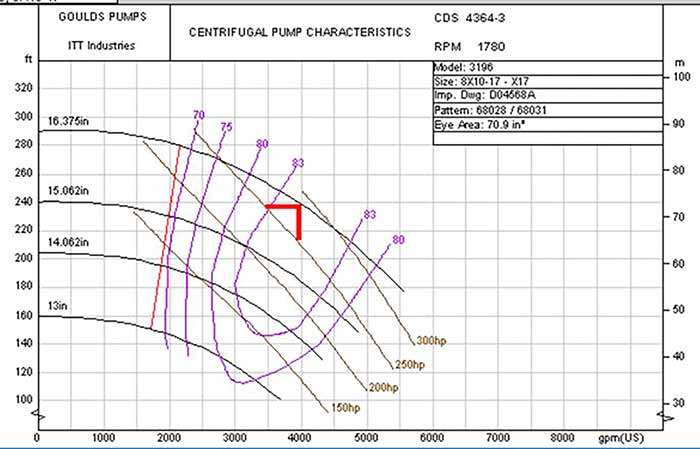
Let’s assume this pump operates at 2,000 gpm (280 feet head), instead of a peak point of 4,000 gpm. The efficiency at the actual operating point is only 70 percent instead of the potentially achievable 83 percent by this pump.
The hp at the operating point is roughly 225 hp (168 kW), and the yearly energy bill is in Equation 5—now, we begin to see some real cost issues!
168 x 24 x 360 x 0.07 = $101,516
At restored efficiency, this would be: 101,516 x (70/83) = $85,616
The net savings would be: 101,516 – 85,616 = $15,900
Equation 5
For larger sizes, the energy savings could be even greater.
As you can see, the net savings depend on how far back away from the BEP the pump operates. Unfortunately, this problem exists in too many actual field installations. Many pumps, procured and installed years ago, no longer operate at the originally intended hydraulic conditions. As operating conditions change, the pump is simply throttled further and further away from the BEP. The result? Dollars “burned,” not to mention other problems (high loads, shaft breakage, etc.).
Obtaining a smaller pump is not a good answer. First of all, a smaller pump usually does not have the hydraulics sized to hit the operating point “dead on.” It may help somewhat, but is expensive and not as efficient. The user’s choice is limited only to the pump sizes available, as standard, from the pump manufacturer’s catalog. Even with a large number of sizes in the catalog, it is virtually impossible to cover each and every variation of the operating conditions. More importantly, however, is the issue of economics and feasibility of piping change, to accommodate a proposed pump downsizing. Piping changes can cost more than a pump.
A better solution is to have a new impeller custom designed and sized for your operating conditions. By doing that, pump performance will essentially shift or slide to exactly where the BEP is, and the net losses become zero. This approach is effective, and the investment is minimal—with a payback of less than a year and often just a few months.
Not only ANSI single-stage overhung-impeller pump designs can benefit from this approach. Cooling water between-bearing pumps are known to have benefited greatly with improved impeller hydraulics. When a metal impeller is replaced with structural engineered composite material (80 percent lighter then metal), the combined effect of hydraulic fine-tuning with reduced weight (and thus load) can be dramatic. Rotordynamic benefits of such an approach are obvious and savings immediate. Other pump types—such as vertical multistage river intake pumps, condenser, circulating, etc.—can have similar issues, and could be likewise retrofitted with improved hydraulics design—quickly, efficiently and economically. More on this in the next edition of our column.
In the meantime, if you suspect that your pump is not operating at the optimum conditions, send us your hydraulic curve and indicate the desired operating condition. We will evaluate the potential energy savings, as a function of your operating conditions in relation to the actual pump BEP. We will then evaluate the costs and impact of rotordynamics, and will provide our engineering recommendations. You may be surprised by how much money you may potentially save.