Centrifugal pumps are a popular choice in many plants because they are simple and reliable, and have a light-weight and compact design. Increased use of centrifugal pumps in many applications, such as process applications, in recent decades has occurred for four reasons:
- Advances in the centrifugal pump seal technology
- Modern hydrodynamic and rotordynamic knowledge and modeling
- Advanced manufacturing methods to produce accurate rotating parts and complex components with reasonable costs
- The ability to simplify the control through the use of modern control technology, particularly modern variable speed drives (VSDs)
Centrifugal pumps do not experience the internal shaking and complex pulsation problems of reciprocating pumps. Therefore, they do not need the same large foundation or have the everyday pulsation problems and component repairs. As plant sizes increase, the pressure to improve reliability is high because of the large economic impact of unplanned downtime. In many large process plants, the impact of nonscheduled shutdowns is much greater than the long-term impact of a small decrease in efficiency (this refers to the lower efficiency of centrifugal pumps compared to positive displacement pumps).
Pump Configuration
Horizontal split casing is commonly used for low- and medium-pressure applications. Large numbers of horizontal split-case centrifugal pumps are installed in petrochemical plants, refineries, water treatment plants and other process plants.
Horizontal split-case pump maintenance is simple and straightforward. To maintain a proper joint seal when the pressure is high, a vertically split (barrel type) pump is used. This article focuses on horizontal split-case centrifugal pumps. Cast iron could be used for low-pressure pumps. For flammable or toxic process liquid services, a suitable steel grade is the minimum requirement. For pump casings, cast steel or fabricated casing should be used. Casings may also be heat treated depending on the thickness, fabrication details, applicable codes and the pump service.
When applications are complex and cannot be accommodated by a single-case pump, multiple cases can be used. A popular configuration is the tandem-driven series arrangement using a common driver. Gear units may be included in a pump train, either between the casings or between the driver and the pump casings. The maximum number of pump casings is usually three. Longer, tandem-driven connected pump trains tend to encounter specific speed problems.
A double-flow pump arrangement could be used for some applications. At the inlet, the liquid stream is divided into parallel steams, and the volume is reduced to a value within the specific capability of a single-flow pump. The pump casing bolts require attention. Proper pre-loading of the casing bolts is necessary to prevent unloading because of the cyclic operation.
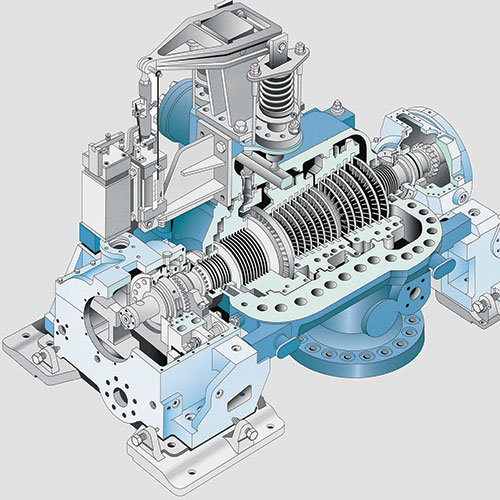
Component Design
Pump shafts should be made from one piece of heat-treated, forged low-alloy steel, suitably ground (forged as close as possible to the final dimensions). Forged low-alloy steel shafts are standard shafts for large centrifugal pumps in process industries. Only pumps that handle highly aggressive liquids require corrosion-resistant shafts. Shaft sleeves are frequently fitted so that the sealing elements do not operate directly against the shaft.
Two types of impellers—a closed impeller (consisting of a hub, blades and a cover) and a semi-open impeller (consisting of a hub and blades) are commonly used. Open impellers are available but rarely used.
The smallest possible pump that meets the application’s specifications is usually preferred. This leads to impellers with high flow coefficients. For a multistage pump, the first stage impeller should have the maximum flow coefficient. In multi-casing, high-pressure pump trains, the first pump casing—because it has the highest suction volume—will dictate the train speed. This will lead to sub-optimum designs as the suction volume of the later stages decreases (particularly in high-pressure services in which the liquid behaves like a compressible fluid). The solution could be a gear unit between the casings for large pressure ratios. This is an advantage of using a higher shaft speed at higher pressures (where the volumetric flow is smaller).
The head generated is fixed by the pump impeller’s dimensions—particularly by the exit angle, tangential tip speed and the slip. The head at the delivery, because of internal losses, also depends on the flow rate. However, the head required is determined by the process conditions (downstream facilities). Identifying all the process duties to be met by a centrifugal pump before the pump order (the pump selection and the design freeze) is important. Once the pump’s design and dimensions are fixed, duties other than those specified may not fall within the pump operating range or can only be accommodated, if at all, by inefficient operation.
For a minimum capital cost, the maximum permissible tip speed is often selected. However, this may lead to a narrow operation range. A wider range and a higher efficiency can be obtained if the tip speed is slightly reduced (5 to 15 percent). The number of impellers needed to achieve a given head could be increased by the reduction of the tip speed, but this solution is preferred.
Manufacturers generally use standard designs arranged in a series of shapes and sizes. Each family of pump impeller covers a range of flow coefficients. The number of impellers that can be accommodated in one pump casing is dictated by rotordynamic considerations.
In the past, oil-lubricated gear couplings were favored for large pump trains. More recently, flexible, dry-element couplings (mainly high torsional stiffness couplings) are replacing gear couplings. Some pump manufacturers may prefer solid couplings or torsion rods between a driver and a pump. The rigid connection (a solid coupling or a flange-to-flange connection) could have the advantage of eliminating a high-speed thrust bearing. However, obtaining the correct alignment may be difficult.
The optimum selection is a diaphragm coupling or a disc coupling. Special care is needed for a coupling guard. The energy stored in a pump rotor assembly is relatively low (with respect to a relatively thick pump casing—good casing thickness), so even if a rotor or part of it splits, the fragments would not penetrate the pump casing. This does not apply to a pump coupling. If a high-speed coupling fails, fragments could penetrate the coupling guards. Special care is, therefore, required when selecting, inspecting and operating couplings and coupling guards with high-speed pumps. The casing of high-speed centrifugal pumps with pressures below 20 bar (gauge) may need a dynamic fragment containment analysis.
Process and Performance
If the pressure ratio is identified precisely, approximately a 9- to 12-percent margin of the pump flow is recommended. If the pressure ratio is a function of the flow (for example, in some recycle applications), around a 5- to 7-percent margin of the flow and pressure is a good suggestion. Higher margins can only be justified if the operation and the capital cost increase are acceptable. In some cases, extra margins are included in the anticipation of future increases in production. This may be an economic solution if VSDs are used.
The centrifugal pump performance curve is head versus flow. For reliable operation, the head curve should rise continuously, at least 8 percent (preferably 10 percent or more) from the certified operating point to the actual shutoff point. For pumps operating in parallel, the head at the same specific flow rate is needed within 3 percent (preferably 2 percent) at any flow rate on the curve.
At flows greater than the rated/design flow, the curve is limited by a rapid fall of the pump head. This is because of high losses, particularly in the front stages of the pump, caused by high liquid velocities and incidence angles at the entry into the impellers. Limiting the flow to 125 percent to 120 percent of the best efficiency point (BEP) is reasonable, preferably with the head above 70 percent to 80 percent of the rated head.
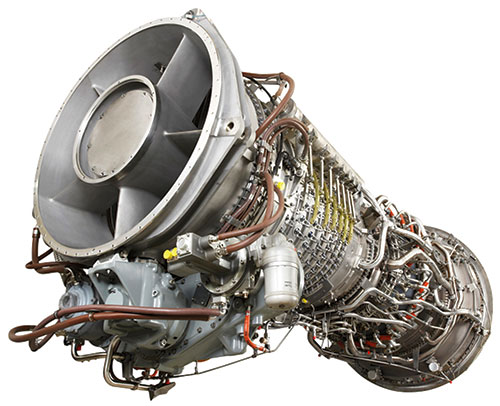
Pump Driver
Many years ago, one of the popular drivers for a centrifugal pump was a steam turbine. Reliability, simplicity and operational convenience were primary factors in the pump driver selection before the increase in energy costs. During that time, a steam turbine—with its ability to operate over a wide speed range and speed match with a pump—was an ideal driver for a centrifugal pump. This choice is still common for large centrifugal pumps in some plants (petrochemical plants, refineries and boiler feed systems). Generally, standard, compact and reliable steam turbine designs (with reasonable cost) are available for use as mechanical drives.
Electric motor drives may require that a gear unit be used. Because fossil fuels can more efficiently be converted to electricity in large central power plants, electric motor drives began to displace steam turbines. Large electric motor drivers using a VSD are popular in plants. The initial cost may prevent the universal acceptance of VSDs for some small pumps. However, a VSD is the most common solution to increase efficiency (particularly at part-load operation) in medium and large pumps.
Electric motors, with a VSD or without, are either induction or synchronous in design. The motor size and the plant electric system requirements determine the parameters for the selection. Synchronous motors are normally only used with very large pumps, with the individual plant specifications influencing the minimum size of the synchronous machine.
Electric motors should conform to the appropriate standards for the application—such as American Petroleum Institute (API) 541 or API 546, or for small motors, Institute of Electrical and Electronic Engineers (IEEE) 841. For electric motors, special considerations should be given to the starting conditions and the effects of pulsating torque. Also, the interaction between the transmission system and the gear unit should be taken into account when VSDs are used.
A gas turbine could be selected as large pump driver based on the available fuel and the specific requirements. Gas turbines are relatively standardized even though they cover a wide range of power and speed. They are not customized to a specific pump application for the power/speed match. Speeds of gas turbines are standard for a given frame/model. Sometimes, the output speed of a gas turbine can be considered when designing an efficient large pump train. If not, an intermediate gear unit will be needed. This adds the complication of another piece of equipment, a higher capital cost and potentially decreased reliability. This gear unit also has a high pitch line velocity. If exhaust heat recovery or regeneration is used, the efficiency of a gas turbine can be an attractive alternative.
Generally, drivers should be sized to continuously deliver no less than 110 percent of the maximum power required by a centrifugal pump. Sometimes, particularly with a gas turbine driver or critical train, this margin may be increased to 12 to 15 percent. Providing a suitable condition monitoring system and a combined control for both the driver and the pump is necessary.
Conclusion
Advanced technologies have been used to provide maintainable, flexible and compact centrifugal pumps. A good driver option for variable speed operation is a steam turbine in the direct drive arrangement or an electric motor with a VSD. Gas turbine applications are limited to special services, sizes and plants—particularly for applications in which cheap fuel is available.