Editor's Note: This is the fourth in a series of five articles based on the Hydraulic Institute's new Positive Displacement (PD) Pumps: Fundamentals, Design and Applications e-Learning course. To read the previous article, click here. To read the next article, click here.
Last month, we introduced Module 3 of the course, which focused on rotary pumps. In this issue, we will focus on Module 4, reciprocating pumps. For the reciprocating pump family, this article will provide an overview of their characteristics, types, application analysis and pump selection process.
Reciprocating Pump Overview
Since the third century B.C. when an Alexandrian named Ctesibus built a basic hand pump that could transfer water, reciprocating pumps have played a significant role in the development of the modern world. Today, reciprocating pumps have developed into technically advanced machines capable of delivering more than 40,000psi of fluid pressure.
Reciprocating pumps comprise a major segment of the positive displacement technology category. Reciprocating pump designs can pump a full range of liquid types including low viscosity chemicals, high particle content slurries and high viscosity materials. Given this wide operating range, they are often the technology of choice for difficult applications.
One main difference between rotodynamic and reciprocating pumps is that for a given speed, the rate of flow of a rotodynamic pump can be varied from zero flow to a maximum flow. Conversely, reciprocating pumps will have a constant flow for a given speed. Pumps that belong to the reciprocating pump family have several common operating characteristics, including a constant fluid delivery per stroke and mechanical trapping of fluid using suction and discharge valves.
Inherent to their reciprocating motion, these pumps also typically produce pulsation. Consideration for additional devices to reduce pulsation, such as pulsation dampeners or attenuators, may be needed for some applications. Additionally, as with many PD pump types, systems may require overpressure relief protection. Efficiencies of reciprocating pumps vary widely across the category due to driver types and mechanical configurations.
Types of Reciprocating Pumps
Four common types of reciprocating pumps-power pumps, power diaphragm pumps, air operated diaphragm pumps and air operated piston pumps-are reviewed in the reciprocating pump module.
Power pumps are reciprocating machines where plungers or pistons are driven within a valved cylinder by a power end. The power end (see Figure 1) converts the rotary motion of a motor-electric, air or hydraulic or diesel engine-into reciprocating motion by means of a crankshaft, connecting rods and crossheads. The liquid end (see Figure 2) connects to the power end and contains the plungers, packing, fluid chambers and valves.
The reciprocating motion of the plunger in concert with the other liquid end components can develop more than 40,000psi of fluid pressure or more than 4,000gpm of fluid flow. These pumps typically have one, two, three, five, seven or nine connecting rods and crossheads that drive an equal amount of fluid plungers. Configurations of an odd amount of cylinders are preferred to reduce pulsation. Pulsation is generated by the oscillating pressure of each fluid chamber from suction pressure to discharge pressure. The oscillating pressure, cycling at 50- to 500-rpm, is a popular driver of pump failures, but these pumps are constructed robustly to resist fatigue.
It is important to control pressure with power pump systems. As the pump injects the displaced liquid into the discharge system, the pressure is increased. The pressure will continue to increase until it meets the requirement of the system. However, if the system pressure requirement is not controlled, the pressure will continue to build up until something ruptures in the system or the pump or the driver of the pump stalls out. Power pumps should be equipped with a pressure relieve device to prevent the over-pressurization of the system beyond its recommended limits.
Power pumps are typically used for low viscosity chemicals, oils, high pressure cleaning, ore slurries, drilling mud, reverse osmosis, saltwater injection, hot oil applications, blow out preventers and subsea applications.
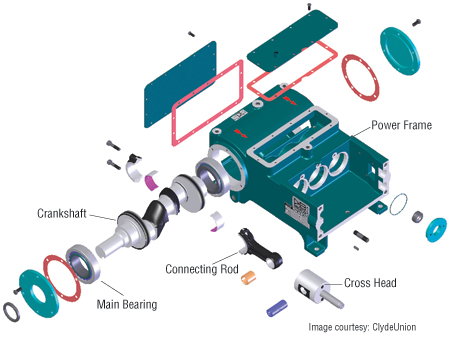
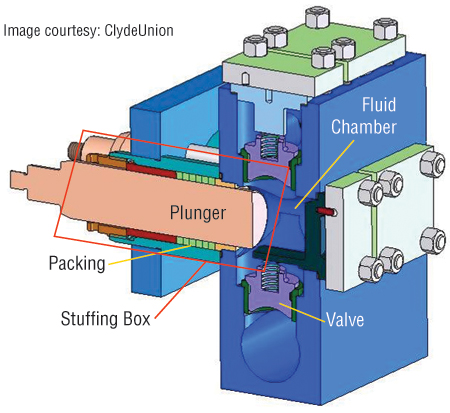
Air operated diaphragm pumps combine the reciprocating motion of a flexible membrane, called a diaphragm, with check valve mechanisms to transfer fluid. These pumps typically have two diaphragms connected to a reciprocating shaft in which one side of the diaphragm is in contact with the liquid being pumped and the other side is in contact with the compressed air. The reciprocating motion is created by an air motor that alternates pressurization of the two diaphragms. Fluid pressure is usually equivalent to the supply air pressure of the pump; however, amplification devices allow specialized pumps to operate at pressures up to three times the supply pressure.
Diaphragm pumps are available in many materials of construction, have no rotating seals, are physically compact and require no electricity connected to the pump for operation (see Figure 3). These characteristics make air operated diaphragm pumps ideal for the transfer of corrosive and abrasive fluids and operation in volatile environments, installations where space is limited and portability is required. Other benefits of these pumps are the ability to self prime, run dry and pass solid entrained fluids.
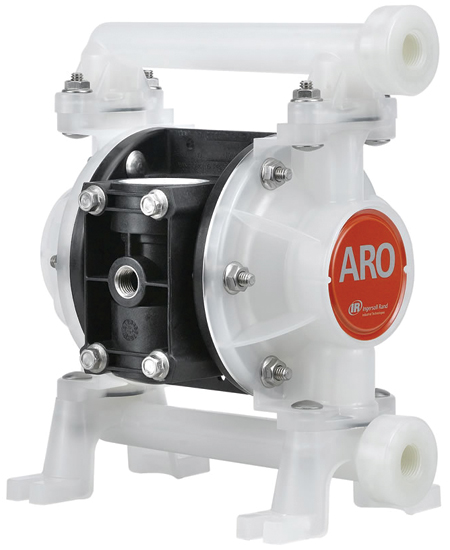
Air operated piston pumps use a reciprocating piston and check mechanisms to produce fluid flow and pressure. As they have the ability to produce fluid pressures in excess of 6,500-psi, these pumps are popular for pumping viscous fluids such as sealants, adhesives, inks and lubricants. Piston pumps typically meet the needs of unique applications that require equipment designed for a particular dispensing, metering or fluid transfer job. As a result, piston pumps are built in many styles and configurations to serve specific needs of users.
The air motor and lower end (see Figure 4) are the two major components that control the flow rate and pressure of the pump. The air motor converts compressed air into a reciprocating motion and is available in several sizes that are defined by the diameter of the air piston. The air motor consists of a major piston connected to the lower end. Driven by the air motor, the lower end uses the reciprocating motion of a smaller diameter piston to create fluid pressure and flow. The ratio of the larger area of the air motor piston to the smaller lower end piston allows for the amplification the fluid pressure over that of the supply air pressure. For example, if the pump is being operated by 100-psi supply air and the air motor piston is four times the area of the lower end, then the fluid pressure would be 400-psi.
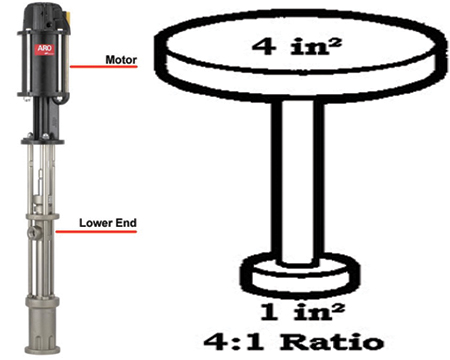
Power diaphragm pumps are designed to combine important advantages of reciprocating plunger and diaphragm pumps. This is done by providing two separate liquid paths, one for the fluid being pumped and one for hydraulic fluid that pressurizes the pumping fluid (see Figure 5). This eliminates the dynamic seal in the pumped fluid loop required with reciprocating plunger pumps and enables leak free operation.
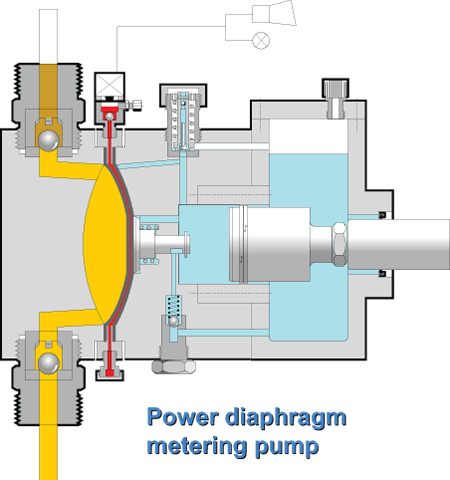
A diaphragm separates these two fluid circuits; suction and discharge valves control the flow in the pump fluid circuit. The diaphragm is the pumping element and its reciprocating motion is induced by the plunger displacing hydraulic fluid. Pumped liquid leakage can occur if a diaphragm is ruptured, but this is prevented by the use of multilayered diaphragms with integral alarm functions. These features allow the fluid to be contained in the pump even when one diaphragm is damaged.
Power diaphragm pump designs are modular, allowing for electric motor driven power frames with multiple pumping heads. Configurations can be simplex, duplex, triplex (see Figure 6), quadrauplex or septuplex. In multi-head configurations, common suction and discharge manifolds provide a reduction in pulsation due to partially overlapping flows. Standard pump configurations are available from manufacturers; however, highly customized units are common with special valves, heating/cooling jackets and wetted parts materials such as titanium, duplex steel or Hastelloy.
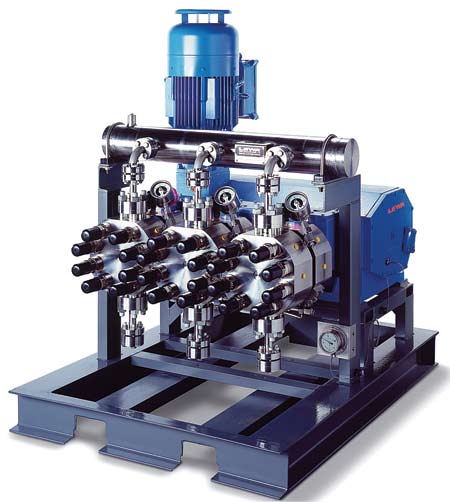
Power diaphragm pumps provide efficient leak free pumping to 1,200-bar (18,000-psi) on a broad range of fluids including fluids that are environmentally damaging, valuable, abrasive or viscous (up to 250-kcSt). These pumps have proven their safety and reliability in numerous applications including chemical process, nuclear, pharmaceutical, cosmetics, biological processes, foodstuffs, and onshore and offshore petrochemical installations.
Pump Selection and Application Analysis
Pump selection is a complex and highly technical process that should be completed by a trained professional. The reciprocating pump-training module addresses the individual considerations required for selection of each type of reciprocating pump. There are some common guidelines for specifying a reciprocating pump for an application. Three common categories requiring consideration are fluid properties, environmental conditions and system requirements.
The fluid being pumped is evaluated for viscosity, specific gravity, temperature, solids content and its compatibility with the pump's materials of construction. All components that come in contact with the fluid must resist corrosion including pump casing materials, seals, packing, diaphragms, plungers and valves. The specified materials of construction for the pump are often the main drivers of its cost. Highly corrosive fluids may require more expensive materials like stainless steel, Alloy C, PVDF, PTFE and Viton; more inert fluids can be compatible with less expensive materials like aluminum, steel, synthetic rubber and thermo plastic elastomers.
Similar to fluid properties, environmental conditions can affect the pump's construction. Atmospheric conditions may require special material pump materials to resist corrosion or ensure groundability in volatile environments.
System parameters such as piping configuration, inlet elevation, outlet elevation, power availability, operating pressure and flow rate are used to determine the best reciprocating pump type and size for the application. These system characteristics in combination with the fluid properties allow for validation that the reciprocating pump will be able to prime and deliver the specified amount of flow and pressure. Specification criteria such as net positive suction head available (NPSHA) from the system, net positive suction pressure required (NPSHR) by the pump and discharge head required by the system are influenced by the above parameters.
When the demands required by the system are calculated and understood, then the pump types that best fit the application can be selected using a range chart (see Figure 7). It is often the case that more than one type of reciprocating pump is suitable for an application; it is then up to the preference of the user to assess the unique features and benefits that each technology has for the application.
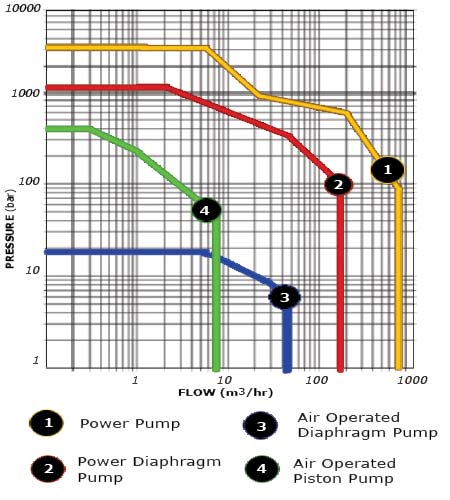
Pumps & Systems, May 2009